Real Time and Linux, Part 3: Sub-Kernels and Benchmarks
In the first two articles of this series [see ``Real Time and Linux'' in the January/February 2002 issue and ``Real Time and Linux, Part 2: the Preemptible Kernel'' in the March/April 2002 issue of ELJ], we examined the fundamental concepts of real time and efforts to make the Linux kernel more responsive. In this article we examine two approaches to real time that involve the introduction of a separate, small, real-time kernel between the hardware and Linux. We also return to benchmarking and compare a desktop/server Linux kernel to modified kernels.
We note and discuss no further that LynuxWorks and OnCore Systems provide proprietary kernels that provide some Linux compatibility. LynuxWorks provides a real-time kernel that implements a Linux compatible API. OnCore Systems provides a real-time microkernel that provides Linux functionality in a variety of ways. These allow one to run a Linux kernel, with real-time performance of its processes, on top of their microkernel.
In this article we concern ourselves primarily with single-CPU real time. When more than one CPU is used, new solutions to real time are possible. For example, one may avoid system calls on a CPU on which a real-time process is waiting. This avoids the kernel-preemption problem altogether. One may be able to direct interrupts to a particular CPU and away from another particular CPU, thus avoiding interrupt latency issues. All of the Linux real-time solutions, incidentally, are usable on multi-CPU systems. In addition, RTAI for example, has additional functionality for multiple CPUs. We are focused, however, on the needs of embedded Linux developers, and most embedded Linux devices have a single general-purpose CPU.
What Is a Real-Time Sub-Kernel?
A typical real-time system has a few tasks that must be executed in a deterministic, real-time manner. In addition, it is frequently the case that response to hardware interrupts must be deterministic. A clever idea is to create a small operating system kernel that provides these mechanisms and provides for running a Linux kernel as well, to supply the complete complement of Linux functionality.
Thus, these real-time sub-kernels deliver an API for tasking, interrupt handling and communication with Linux processes. Linux is suspended while the sub-kernel's tasks run or while the sub-kernel is dealing with an interrupt. As a consequence, for example, Linux is not allowed to disable interrupts. Also, these sub-kernels are not complete operating systems. They do not have a full complement of device drivers. They don't provide extensive libraries. They are an addition to Linux, not a standalone operating system.
There is a natural tendency, however, for these sub-kernels to grow in complexity, from software release to software release, as more and more functionality is incorporated. A major aspect of their virtue, though, is that one may still take advantage of all the benefits of Linux in one's application. It is just that the real-time portion of the application is handled separately by the sub-kernel.
Some view this situation as Linux being treated as the lowest priority, or idle, task of the sub-kernel OS. Figure 1 depicts the relationship of the sub-kernel and Linux.
The sub-kernels are created with Linux by doing three things: 1) patching a Linux kernel to provide a few hooks for things like added functionality, 2) modifying the interrupt handling and 3) creating loadable modules to provide the bulk of the API and functionality.
Sub-kernels provide an API for use by the real-time tasks. The APIs they provide resemble POSIX threads, other POSIX functions and additional unique functions. Using the sub-kernels means that the real-time tasks are using APIs that may be familiar to Linux programmers, but they are separate implementations and sometimes differ.
Interrupt handling is modified by patching the kernel source tree. The patches change the functions, for example, that are ordinarily used to disable interrupts. Thus, when the kernel and drivers in the Linux sub-tree are recompiled, they will not actually be able to disable interrupts. It is important to note this change because it means, for example, that a driver compiled separately from these modified headers may actually disable interrupts and thwart the real-time technique. Additionally, nonstandard code that, say, simply inlines an interrupt-disabling assembly language instruction will likewise thwart it. Fortunately, in practice, these are not likely situations and certainly can be avoided. They are examples to reinforce the idea that no real-time solution is completely free from caveats.
RTLinux and RTAI
The two most commonly used sub-kernels are RTLinux and RTAI. Both RTLinux and RTAI are designed for hard real time. They are much more (and a little less) than just a preemptible kernel. In practical terms, a real-time operating system provides convenience to developers. RTLinux and RTAI provide a wealth of additional, real-time, related functions. RTAI, for example, provides rate-monotonic scheduling and early-deadline-first scheduling, in addition to conventional priority scheduling.
The sub-kernels provide both POSIX and proprietary functions, as well as functions to create tasks, disable/enable interrupts and provide synchronization and communication. When using RTLinux or RTAI, a developer uses a new API in addition to their POSIX functions.
Both RTLinux and RTAI furnish some support for working with user-space processes. This is important because a real-time application for Linux naturally will want to make use of the functionality of Linux. RTLinux provides support for invoking a signal handler in a user-space process, in addition to FIFOs and shared memory that can be read and written in both kernel and user space. RTAI provides FIFOs, shared memory and a complete, hard real-time mechanism, called LXRT, that can be used in user space.
These mechanisms, though, don't make the Linux kernel real time. A user-space process still must avoid system calls because they may block in the kernel. Also, it seems neither RTLinux nor RTAI have been enhanced to work with a preemptible kernel. Since the two approaches are both beneficial, and mostly orthogonal, perhaps they will be combined in the near future. This may be likely since the Love patches are now part of the standard 2.5 kernel tree and perhaps will be a part of the stable, 2.6 kernel whenever it is released.
Some Thoughts on the Choices
For a developer requiring real-time enhancements, choosing among RTLinux, RTAI, the Love preemptible kernel and the TimeSys preemptible kernel, there are a myriad of issues. Let's highlight a few that many developers value.
-
Which are maintained in an open-source manner where independent outsiders have contributed? RTAI and Love.
-
Which have a software patent for their underlying technique? RTLinux.
-
Which are part of the 2.5 Linux kernel tree? Love.
-
Which have additional real-time capabilities besides preemptibility? TimeSys, RTAI and RTLinux.
-
Which are positioned such that one can reasonably assume that the solution will continue to be freely available for free download? RTAI and Love (in my humble opinion).
-
Which give control over interrupts and are likely to provide near-machine-level resolution responsiveness? RTLinux and RTAI.
Kernel Availability for Different Processors
None of these real-time approaches are available for every CPU on which Linux runs; extra effort is required to adapt the solution to a new processor. But, as the four solutions we examine here have quite active development, it is safe to assume that support for additional CPUs is at least contemplated.
As a snapshot, the Love preemptible kernel is currently only available for x86, but with MontaVista's support it is likely to be ported to most, if not all, of the CPUs that MontaVista supports. That includes PowerPC, ARM, MIPS, SuperH, etc. The TimeSys kernel is currently available for PowerPC, ARM, SuperH and Pentium. RTLinux is available for x86 and PowerPC. RTAI is available for x86 and PowerPC.
Benchmarks
You may download the benchmark programs from the Web (see the Resources Sidebar under K Computing Benchmarks). All of our benchmarks were run on a 465MHz Celeron. Other x86 CPUs, however, have produced similar results. We have not benchmarked on other kinds of CPUs.
We benchmarked the Red Hat 7.2 kernel, which is based on Linux kernel 2.4.7; the TimeSys Linux 3.0 kernel, which is based on Linux 2.4.7; and a kernel patched with Robert Love and MontaVista's preemption patch for Linux kernel 2.4.18. We will refer to these kernels as Red Hat, TimeSys and Love, respectively. We separately benchmarked the RTAI and RTLinux kernels.
The benchmark consisted of timing the precision of a call to nanosleep(). Sleeping for a precise amount of time closely relates to the kernel's ability to serve user-space, real-time processes reliably. The Linux nanosleep() function allows one to request sleeps in nanosecond units. Our benchmark requests a 50 millisecond sleep. Interestingly, a request to nanosleep() to sleep N milliseconds reliably sleeps 10 + N milliseconds. Thus, we measure jitter with respect to how close the sleep was to 60 milliseconds. Also, one should note that nanosleep() is a busy wait in the kernel when the request to sleep is for an amount of two milliseconds or less. Therefore, a busy wait would not simulate interrupt response time as well as a true sleep.
The benchmark program takes 1,000 samples. The last 998 are used in the graph. The first two are discarded to avoid cache slowdowns as a result of a cold cache. The benchmark program was locked into memory via mlockall() and given the highest FIFO priority via sched_set_scheduler() and sched_get_priority_max().
The heart of our benchmark is:
t1 = get_cycles(); nanosleep(fifty_ms, NULL); t2 = get_cycles(); jitter[i] = t2 - t1;
The get_cycles() function is a machine-independent way to read the CPU's cycle counter. On x86 machines it reads the timestamp counter (TSC). The TSC increments at the rate of the CPU. Thus, on a 500MHz CPU, the TSC increments 500,000,000 times per second. The frequency of the CPU is determined by examining the CPU speed value listed in /proc/cpuinfo. The read of the TSC takes on the order of about ten instruction times and is extremely precise in comparison to the interval we are timing.
The difference, in milliseconds, from our expected sleep time of 50 + 10 milliseconds for a given value of jitter, is calculated as
diff = (jitter/KHz) - 10 - 50;
The five benchmarks used the stress tests of Benno Senoner, which are part of his latency test benchmark. These tests stress the system by copying a disk file, reading a disk file, writing a disk file, reading the /proc filesystem and performing the X11perf test. The graphs of the three kernels for these loads are shown in Figures 2-6.
Since the Red Hat kernel is clearly much less responsive than the Love or TimeSys kernels, we separately graph just the Love and TimeSys kernel results. These are depicted in Figures 7-11.
It is apparent from the graphs that the preemptible kernels provide significant improvement in responsiveness. Because they represent much improved performance, without a change in the API required to be used by an application, they are clearly attractive choices for embedded Linux developers.
RTLinux and RTAI Benchmarks
One justifiably expects that RTAI and RTLinux will provide rock-solid performance even under great loads. They meet these expectations, evidenced through our benchmarks. One must remember, though, that there are still a few caveats. Some issues to keep in mind that can thwart real-time performance: perform no blocking operations such as memory allocation; don't use any drivers that haven't been patched to avoid truly disabling interrupts, and avoid costly priority inversions.
To benchmark RTAI and RTLinux we created a periodic task and measured its timing performance against the requested periodic rate. The worst-case performance for both RTLinux and RTAI is on the order of 30 microseconds or less. Our benchmark programs are available for free download (see the Resources Sidebar under K Computing Benchmarks).
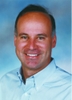
Kevin Dankwardt is founder and CEO of K Computing, a training and consulting firm in Silicon Valley. In particular, his organization develops and delivers embedded and real-time Linux training worldwide.